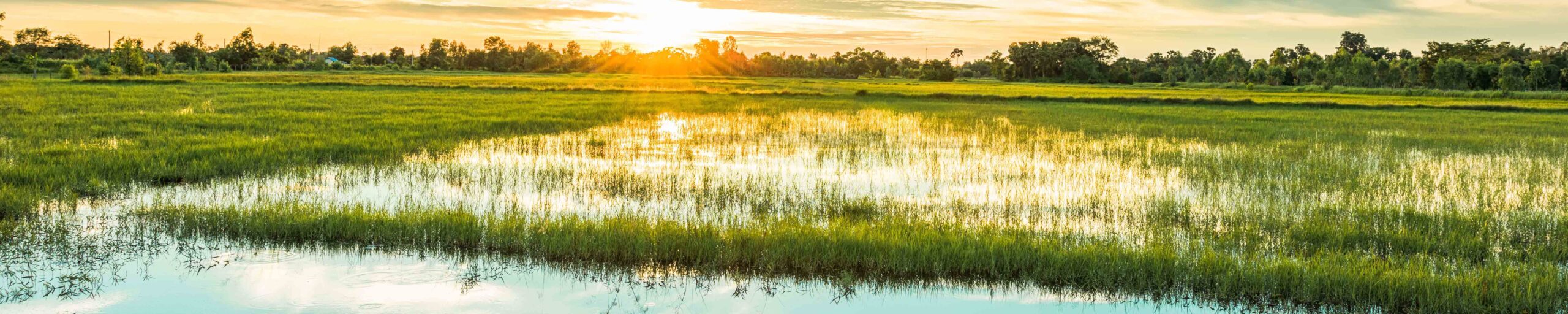
Sustainable
Phosphorus Alliance
Green phosphorus. Blue water.
Your life depends on it
There is no life without phosphorus. It’s in your bones. It’s in your DNA. As a key fertilizer ingredient, phosphorus is essential for global food security.
As a burgeoning world population harvests more crops for food, energy, and materials, the demand for phosphorus increases. Yet our phosphate rock reserves are non-renewable, diminishing in quality and highly concentrated geographically, posing geopolitical vulnerability. Meanwhile, intensified phosphorus use has degraded water quality in rivers, lakes, and coastal oceans, creating toxic algal blooms and dead zones. Our global water security also depends on better phosphorus management.
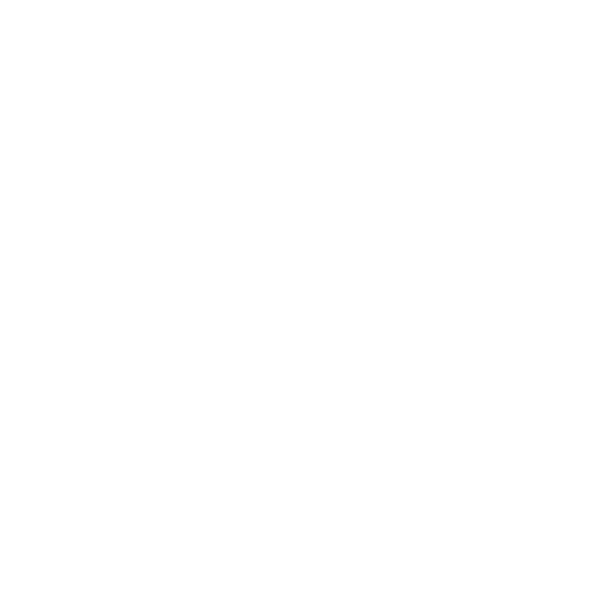
Our Issues
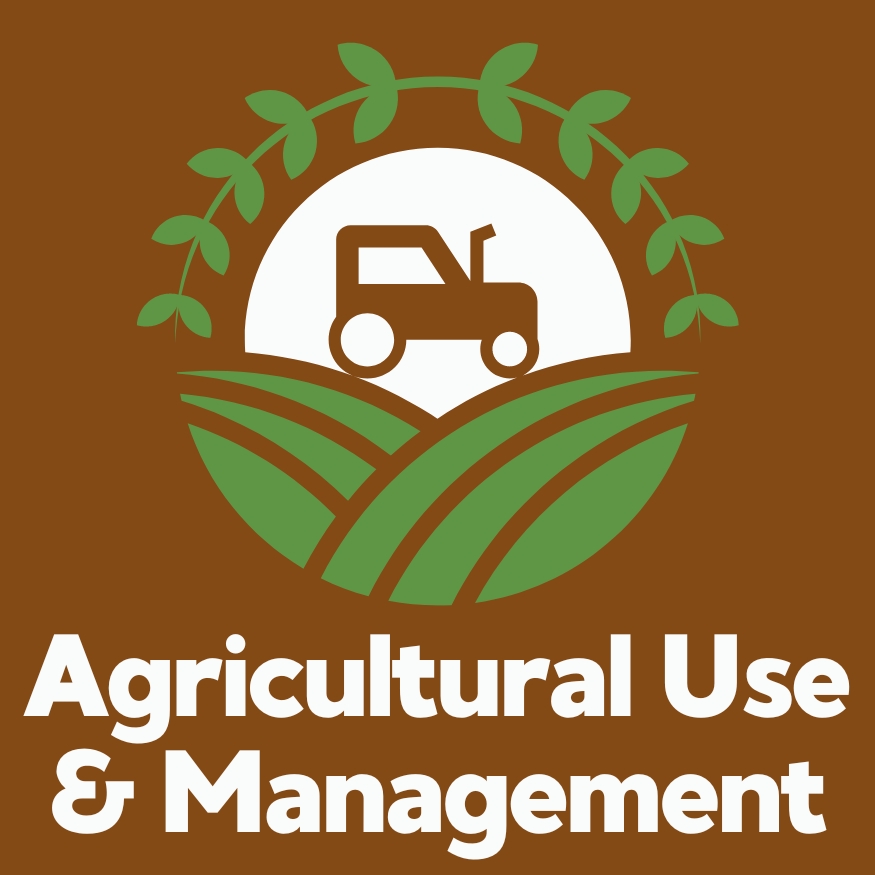
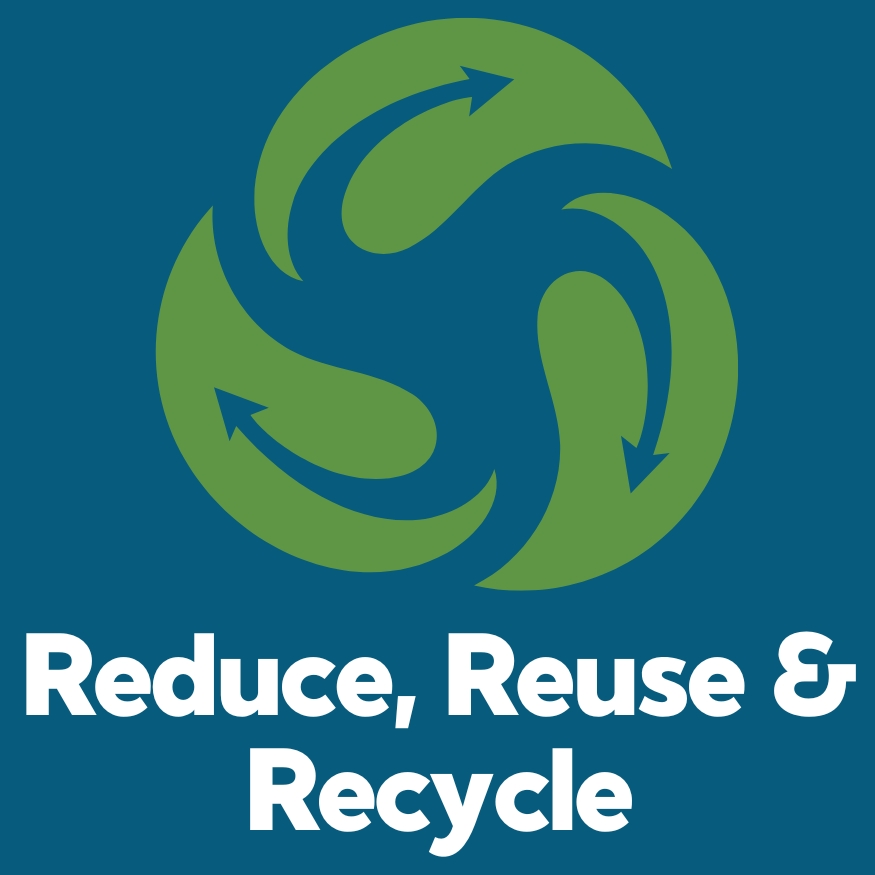
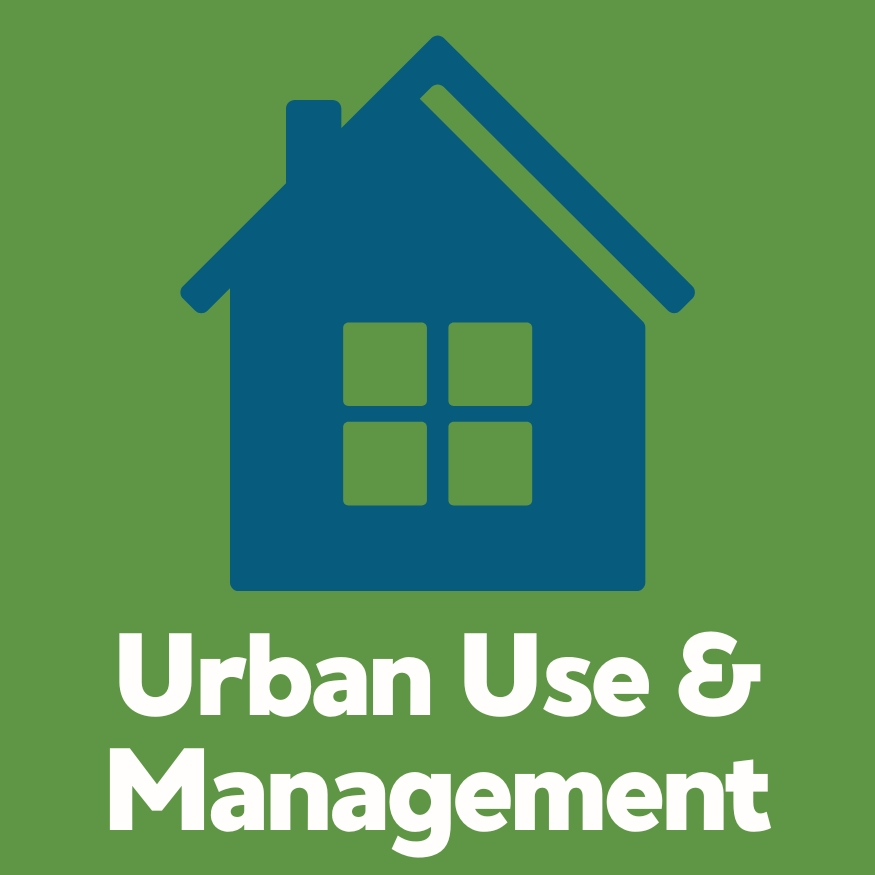
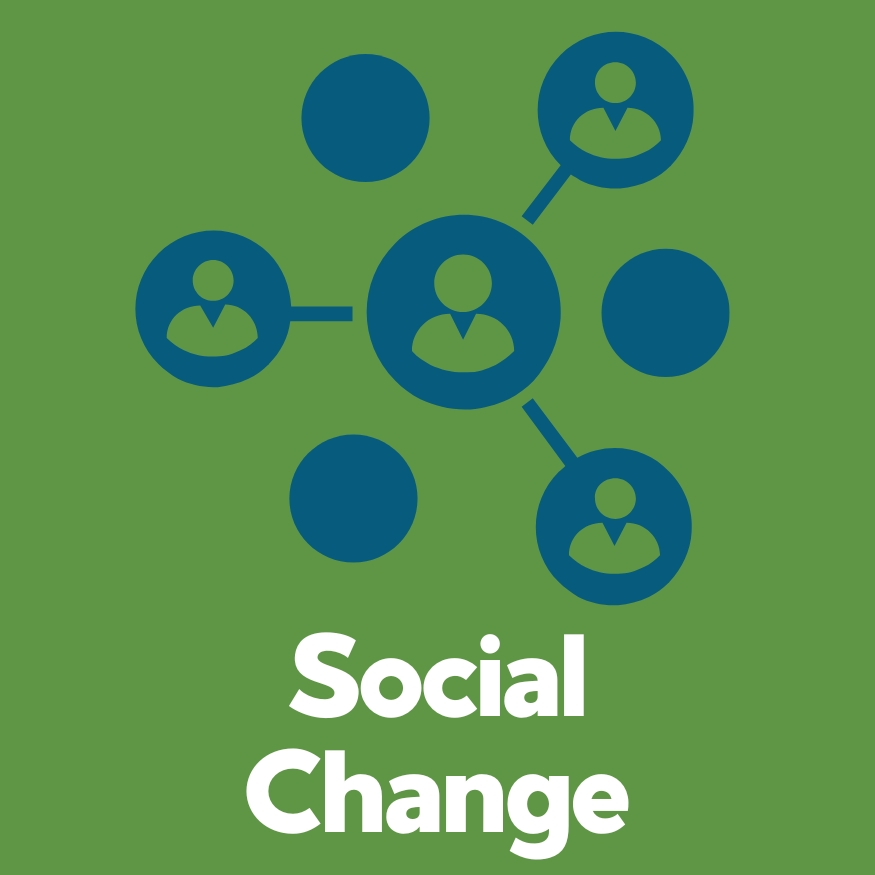
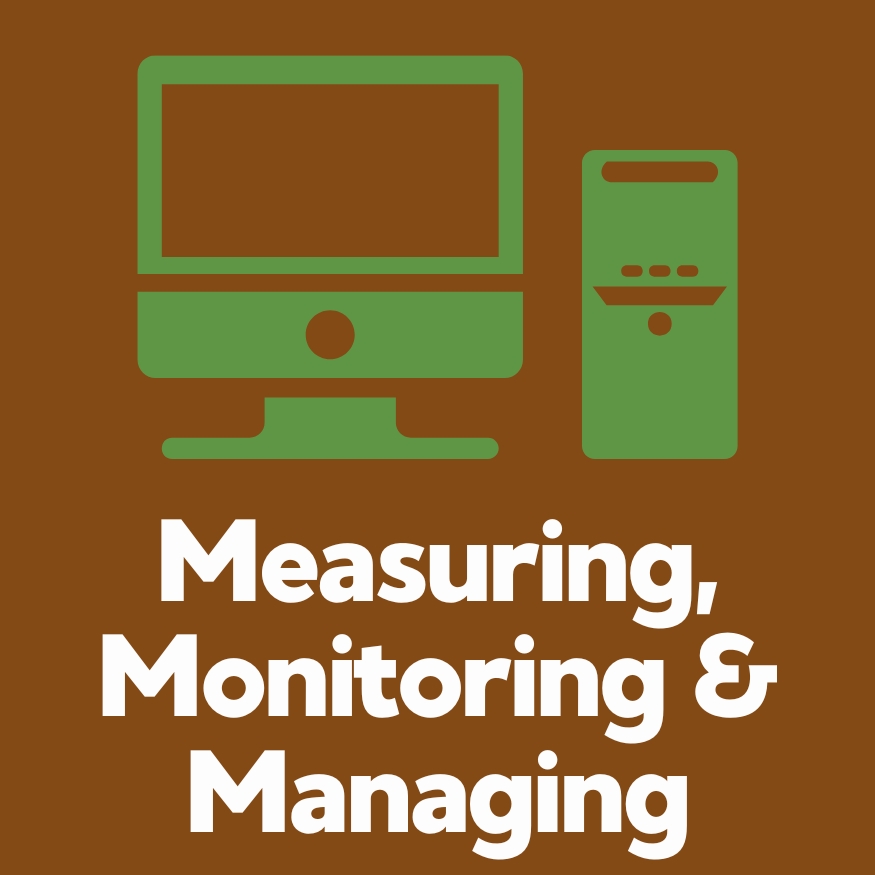
Allies addressing a complex problem
The Sustainable Phosphorus Alliance is a member organization addressing the complex problem of phosphorus sustainability. We are North America’s central forum and advocate for the sustainable use, recovery, and recycling of phosphorus in the food system. We collaborate with our members and supporters to innovate and implement evidence-based solutions to the phosphorus sustainability challenge, to be shared and accessible to the community. Our activities are funded by grants, membership dues, and donations.
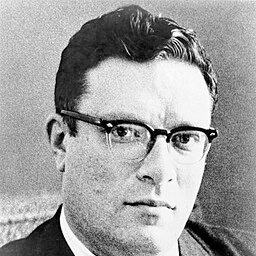
Life can multiply until all phosphorus is gone, and then there is an inexorable halt which nothing can prevent.